How do brains tune in to one neural signal out of billions?
Like a cocktail partygoer able to focus on one discussion in a noisy room, brains are able to make reliable connections against a busy neural background. Here are two phenomena that help it happen.
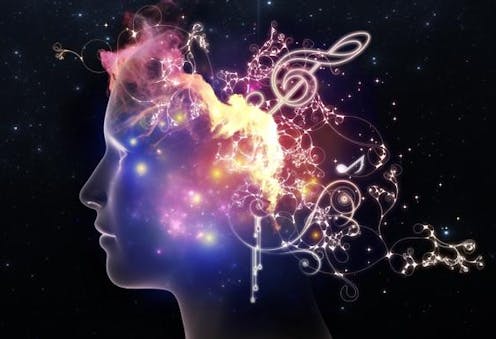
The human brain sends hundreds of billions of neural signals each second. It’s an extraordinarily complex feat.
A healthy brain must establish an enormous number of correct connections and ensure that they remain accurate for the entire period of the information transfer – that can take seconds, which in “brain time” is pretty long.
How does each signal get to its intended destination?
The challenge for your brain is similar to what you’re faced with when trying to engage in conversation at a noisy cocktail party. You’re able to focus on the person you’re talking to and “mute” the other discussions. This phenomenon is selective hearing – what’s called the cocktail party effect.
When everyone at a large, crowded party talks at roughly the same loudness, the average sound level of the person you’re speaking with is about equal to the average level of all the other partygoers’ chatter combined. If it were a satellite TV system, this roughly equal balance of desired signal and background noise would result in poor reception. Nevertheless, this balance is good enough to let you understand conversation at a bustling party.
How does the human brain do it, distinguishing among billions of ongoing “conversations” within itself and locking on to a specific signal for delivery?
My team’s research into the neurological networks of the brain shows there are two activities that support its ability to establish reliable connections in the presence of significant biological background noise. Although the brain’s mechanisms are quite complex, these two activities act as what an electrical engineer calls a matched filter - a processing element used in high-performance radio systems, and now known to exist in nature.
Neurons singing in harmony
Let’s take a moment to focus on just one of the hundreds of billions of nerve fibers in the human brain, of which many are typically active at any given point in time. They’re all doing their part to carry out thought processes that allow humans to function successfully and interact meaningfully with each other – supporting abilities such as orientation, attention, memory, problem-solving and executive function.
My research team has developed a model that translates biological brain activity to the human audible range, so we can hear the brain at work. Here’s what a single nerve fiber transmitting its signal sounds like in an ideal, noise-free environment:
When this chosen nerve fiber transmits a signal to its target destination elsewhere in the brain, it’s up against the background noise caused by the activity of all the other active fibers. Here’s the sound of that same fiber now immersed in the brain’s cocktail party:
The background noise in the brain stimulates a small population of other nerve fibers around our chosen nerve fiber to synchronize and transmit roughly the same message. This synchronization reduces the effect of the noise and improves the clarity of the signal.
It does the job, but is not perfect. It’s similar to many voices singing in harmony. Each voice projects sound at its unique frequencies at each moment, with the sum total of the multitude of voices extending the frequency range of each individual voice. Think of a chorus filling a music hall with its song, as opposed to a soloist singing just one part. This strategy enriches the frequency content, raises the level of the transmitted signal and increases the quality of the reception.
Scientists describe this phenomenon as the emergence of a relationship, or coupling, between physically separated subsystems of nerve fibers. It creates a larger, dynamical system. The idea is not so different from the 350-year-old mystery, finally solved, of how pendulum clocks mounted on the same wall synchronize through small physical forces exerted on the supporting beam.
My colleagues and I believe that this same ability to “sync up” might lead to the discovery of noninvasive therapeutic treatments for neurological disorders such as multiple sclerosis. This could be accomplished using a noninvasive neuromodulator device at the surface of the scalp to provide small, nonphysical custom electric field forces to the region of the brain affected by the disease. By noninvasively altering the patient’s brain signals, these electric field forces would create a healthier neurological network environment for information transfer.
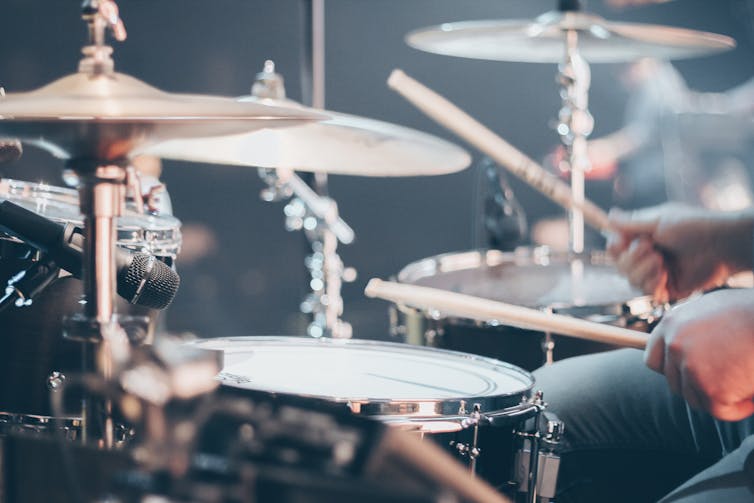
Brains rolling the drums
The second way brains cut through the signal clutter is what neuroscientists refer to as the delivery key. It’s the role played by the brain’s natural rhythms, popularly known as brainwaves.
These brain rhythms are created by nerve cells that fire in specific patterns, causing waves of electrical activity at certain very low frequencies, ranging from about 0.5 to 140 cycles per second. By comparison, smartphones operate at around 5,000,000,000 cycles per second. The waves that help to deliver a signal to a destination in the brain’s noisy environment appear to be either Alpha waves, 8 to 13 cycles per second, or Beta waves, 13 to 32 cycles per second.
In my lab, we refer to this second activity as like “rolling the drums.” The brainwave frequency is similar to that of the sub-bass or bass drum used to mark or keep time in military, rock, pop, jazz and traditional orchestra music.
These low-frequency rhythms act as a delivery key that is impressed on the transmitted signal as an additional frequency. It’s sort of like how GPS signals synchronize telecommunications networks. Say that the brainwave signal or delivery key is 10 cycles per second. The time duration of one cycle is a tenth of a second, so the delivery key gives a time marker at the reception point every tenth of a second.
This time marker is extremely helpful in the accurate reception of the transmitted signal. Crucially, this delivery key only opens, or activates, the lock at the intended reception point. The idea is not so different from the use of a password to gain access to specific content.
Neuroscientists believe that the choice of delivery key used depends on the state of the individual. For example, Alpha waves are associated with wakeful rest with eyes closed. Beta waves are associated with normal wakeful consciousness and concentration.
Scientists suppose that associated with each delivery key, or brain rhythm, is a list of cognitive functions consistent with the individual’s state. So, for example, a signal sent with a 10 cycles per second Alpha wave brain rhythm impressed upon it already has information encoded in it about wakeful rest.
Brainwaves of electrical activity were identified almost 100 years ago, and researchers are constantly learning more about them and their role in behavior and brain function.
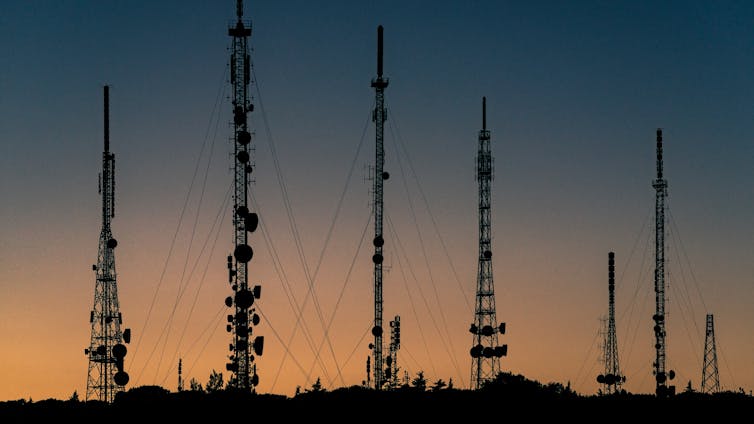
Modeling built systems on the brain
My lab’s research into neurological networks has implications for not only understanding the human brain and developing noninvasive diagnostic procedures and therapeutic treatments for a variety of neurological dysfunctions, but also for designing improved systems for telecommunications, networking, cybersecurity, artificial intelligence and robotics.
For example, the human brain demonstrates just how much more advanced telecommunications network system designs could be. 5G cellular networks hope to serve about 1 million devices in a square mile. In contrast, the human brain can rapidly establish at least 1 million connections within a cubic inch of brain tissue.
Today’s telecommunications network system designs are constrained because they essentially draw from the principles of one discipline – electrical and computer engineering. Even the simplest circuits of the brain, the nerve fibers, which are like the links in a telecommunications network, operate in exceedingly complex ways according to combined principles of biology, chemical engineering, mechanical engineering and electrical and computer engineering.
Designing systems similar in capability to the human brain will require the much more multidisciplinary approach reflected in my research group – a team drawn from experts in medicine, life sciences, engineering and advanced materials – and research partners.
[ Deep knowledge, daily. Sign up for The Conversation’s newsletter. ]
Salvatore Domenic Morgera has received funding for research in networks from the Natural Sciences and Engineering Research Council of Canada, The Fonds de recherche du Québec - Nature et technologies, National Science Foundation, United States Special Operations Command, IBM, Harris Corporation, CMC Electronics, Motorola, Bell Canada, and other public and private agencies.
Read These Next
I study modern-day slavery − and here’s what I’ve learned about how enslavers try to justify their a
To stop a crime, it helps to understand who these criminals are and how they think.
Trump’s push to control Greenland echoes US purchase of Alaska from Russia in 1867
The tale of how and why Russia ceded its control over Alaska to the US 150 years ago is actually 2 tales…