How the zebrafish got its stripes
Zebrafish are known for their black and gold stripes, but researchers are still figuring out how pigment cells interact to form these patterns.
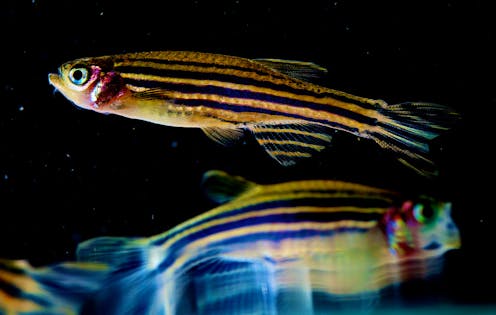
Stripes are common in our lives. It’s a pretty basic pattern, and easy to take for granted.
As an applied mathematician who studies how patterns form in nature, though, I am wowed by the striped patterns the zebrafish wears across its body and fins.
Take a closer look at zebrafish’s black and gold stripes, and you’ll see different-colored pigment cells, tens of thousands of them. I like to envision these cells as people walking around in a crowded room: Just like us, the cells move and interact with their neighbors. Stripes appear because the cells very carefully instruct and signal each other on how to behave. They even “shake hands” in some sense by reaching toward distant cells.
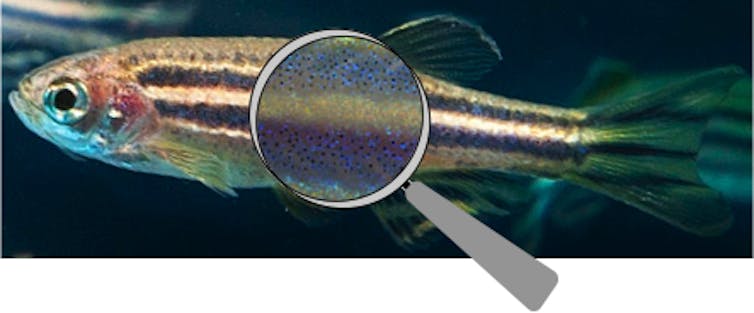
From a mathematical perspective, zebrafish stripes fall into the field of self-organization, a phenomenon in which individuals interact to produce some pattern much bigger than any individual, without external direction. Bird flocks and schooling fish are also examples of self-organization in nature. No one is on a megaphone calling out directions so that birds flock or pigment cells produce fish stripes, yet remarkably, they both organize themselves to create patterns.
Until recently, the research community thought only two types of cells were involved in zebrafish stripes: black and gold stripes, so black and gold cells. However, experiments showed that a third type of pigment cell – blue and silver iridophores – is critical to pattern formation. Remove it from the skin, and zebrafish have spots!
So how do thousands of different-colored cells on a growing zebrafish work together to consistently form stripes? To help answer this question, I developed a mathematical model in collaboration with applied mathematics professor Bjorn Sandstede. In our model, pigment cells are colored dots following prescribed rules and equations for how they move around, interact and change their color. Cells with different colors behave in different ways. There are lots of questions about zebrafish, so we decided to focus on the newcomers to the scene: those pesky blue and silver cells.
Math offers a different perspective from typical biological experiments on fish. Biologists can watch how cells behave, but it’s trickier to deduce the signals behind their behavior. Using mathematical models, we can test lots of different possible cell interactions and suggest which ones are actually able to explain the behaviors biologists observe. Biologists can then test our predictions on real fish.
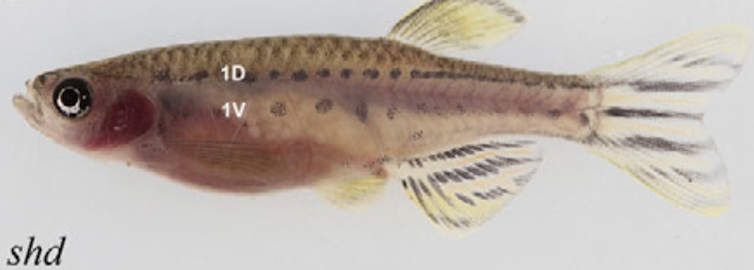
Our model suggests there are multiple signals at work that instruct silver and blue cells on the fish skin. All these signals are redundant. A few cues are all the instruction a cell may need in a perfect world, but the world isn’t perfect. For example, we think that nearby black cells signal iridophores to change their density and color. But if there are not any black cells around to transmit that signal, distant gold cells can fill in and provide the same instructions.
You can think of these redundant signals like a bunch of different alarm clocks. If you have an important meeting in the morning, you may set an alarm clock, put a notification on your phone and ask for a wake-up call. All that redundancy means that you will probably get a bunch of cues to wake up. But on the off chance that your phone dies or the front desk forgets to call, it also means you’ll still get to your meeting on time. The redundancy ensures the desired result, even if one signal fails.
The same idea may be at work in zebrafish. Our model suggests that different-colored cells are constantly instructing each other. This ensures that blue and silver iridophores are pummeled with directions from all sides on how to behave. Because there are multiple signals, occasional failures don’t disrupt patterns too much. The result: reliable stripes.
Why is this important? Zebrafish genes are surprisingly similar to human genes. By understanding how pigment cells interact in normal and mutated zebrafish, researchers may be able to start to link genes to their function.
The story of how zebrafish patterns form isn’t finished yet. For now, though, the next time you see a striped fish, consider pausing a moment to recognize all the work pigment cells put into creating that pattern. Those dependable stripes are pretty darn amazing.
Alexandria Volkening was funded by the Mathematical Biosciences Institute and the National Science Foundation for this study under grants DMS-1148284, DGE-0228243, and DMS-1440386.
Read These Next
More Americans of all political stripes support government benefits for low-income people − and Blac
Disparaging people who get government benefits to make ends meet, especially if they’re Black, is…
The New Yorker turns 100 − how a poker game pipe dream became a publishing powerhouse
The magazine, founder Harold Ross famously declared, would not be ‘edited for the old lady in Dubuque.’…
How populist leaders like Trump use ‘common sense’ as an ideological weapon to undermine facts
When common sense is promoted as a virtue, it’s not just to celebrate how regular people understand…