Sugary handshakes are how cells talk to each other − understanding these name tags can clarify how t
Sugar molecules called glycans cover the surface of all cells, acting as ID cards that broadcast what they are to the rest of the body.
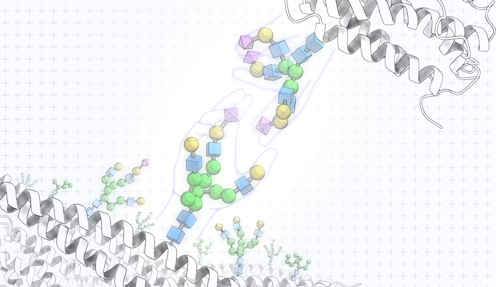
Like the people they make up, cells communicate by bumping into one another and exchanging handshakes. Unlike people, cells perform these handshakes using the diverse range of sugar molecules coating their surface like trees covering a landscape. Handshakes between these sugar molecules, or glycans, trigger cells to react in specific ways toward each other, such as escape, ignore or destroy.
Figuring out the “body language” of glycans during these handshakes can provide clues to how cancers, infections and immune systems work, as well as solutions to health and sustainability challenges society faces today.
What are glycans?
Each glycan molecule is made up of a network of individual sugar molecules bonded together. The vast number of possible glycan structures that can be built from connecting these sugar molecules together allows glycans to store rich information.
Because all living cells are covered with sugars, glycans act like ID cards for cells. They display the cell’s identity, such as whether it’s a bacteria or human cell, and its state, such as whether it’s healthy or cancer, to the rest of the body and allow other cells to recognize and respond to it. For example, these identifying signs allow our immune cells to recognize and clear out harmful bacteria and cancerous cells while leaving healthy cells in peace.
An example of how glycan-stored information is important to daily life is your blood type. Glycans are chemically bonded to proteins and lipids on the surface of red blood cells. Notably, the surface of type A red blood cells have glycans that differ from the glycans on the surface of type B and type O red blood cells. Knowing what blood type you have is important to avoid an unwanted immune response during blood transfusions.
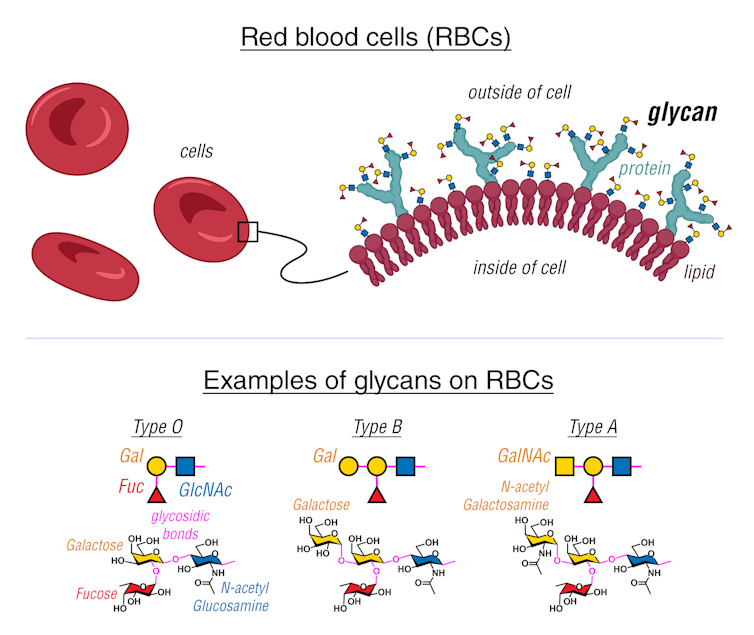
Proteins decorated with glycans, or glycoproteins, and lipids decorated with glycans, or glycolipids, are ubiquitous in nature.
For example, distinctive glycoproteins cover the surface of the viruses that cause COVID-19, HIV and H1N1 influenza and help them infect cells. Glycolipids also coat many bacteria, allowing them to stick to their hosts and protect them from viruses and immune cells.
More recently, researchers discovered pieces of genetic material decorated with glycans on the surfaces of mammalian cells, challenging the long-standing notion that genetic material could be found only in the nucleus of cells and launching research to determine the functions of these glycans. One recent study showed that these molecules are vital in attracting immune cells toward infected or injured tissues.
How do cells read glycans?
In addition to the rich biological information contained in glycans, their easily accessible locations on cell surfaces make them highly attractive targets in scientific research and drug development.
Cells sense glycans on the surfaces of other cells by using proteins called lectins, among others. Each lectin has a unique area that allows it to bind to glycans with a specific matching sequence, triggering complex signals that lead to a biological action.
For example, a subfamily of lectins called C-type lectins are able to recognize the specific glycans on the outer walls of harmful viruses, fungi and bacteria. Found on surfaces of certain immune cells, these lectins deliver the glycans to proteins on other immune cells that can now selectively destroy any viruses or cells that carry that glycan. This process allows the immune system to clear the body of harmful pathogens. For example, these lectins recognize glycans on the surfaces of cancer cells and direct other immune cells to eliminate these cancer cells.
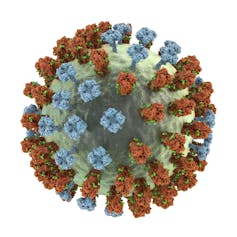
Another type of lectin called siglecs are found on surfaces of immune cells and help them distinguish self from nonself, that is, between the cells that make up the body and the cells that are foreign to the body. Because siglecs are involved in controlling how the immune system responds to many cancers, allergies, autoimmune diseases and neurodegeneration, they offer an opportunity to treat these conditions.
The early success of glycan-based drugs is exemplified by Pfizer’s Prevnar vaccine to prevent bacterial pneumonia, which was approved by the Food and Drug Administration in 2010. Prevnar contains glycans from various strains of Streptococcus pneumoniae, the leading cause of bacterial pneumonia in children and adults. The bacterial glycans in the vaccine trigger an immune response when immune cells recognize the glycans as foreign threats. Once immune cells learn how to neutralize the threat, the body becomes immune to future invasion by bacteria with the same glycans.
Examining every sugar molecule
Because scientists are still unable to extract all the biological information in glycans, their full potential as treatments has remained untapped. Comprehensively extracting all the information stored in glycans is very difficult because there isn’t currently technology able to analyze the complex and diverse structures of glycans. Researchers still don’t know what these “sugar codes” look like and how they function.
Individual glycans are composed of sugar molecules in unique arrangements, but current analytical tools can only simultaneously analyze many glycans. To see why this is a problem for analysis, imagine all the glycans in a cell as candies in a jar. Some of them are the same colors and some are not. It would be difficult to identify and quantify the color of every candy in the jar if you’re unable to pour them out to individually sort through each one of them.
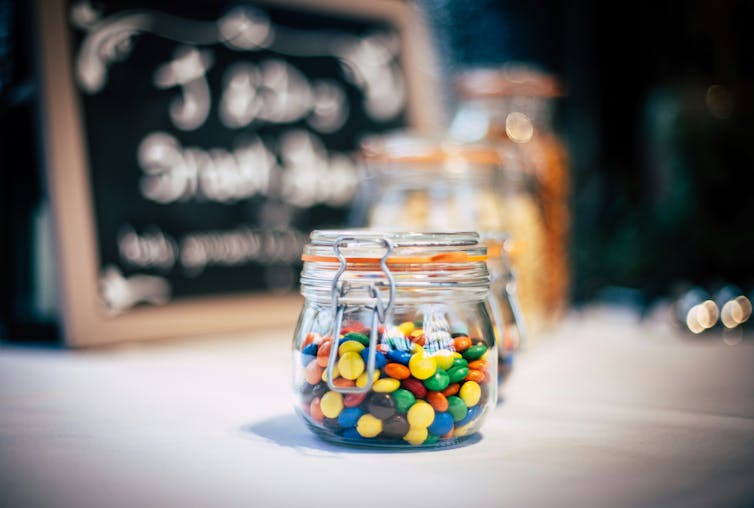
My lab is confronting this challenge by developing imaging technology that can analyze the structure of glycans by imaging each individual molecule. Essentially, we’re developing a technique to open the jar and study every single candy one at a time.
In the long run, my team aspires to unveil how these glycans present themselves to the proteins that recognize them and, finally, reveal the very language that cells use to express themselves.
Kelvin Anggara works for the Max Planck Institute for Solid State Research and receives funding from the European Research Council under Project GlycoX (101075996).
Read These Next
Trump’s promotion of an image of strength after assassination attempt borrows from authoritarian pla
History is dotted with instances of strongmen who burnish their image or crack down on opponents after…
New treatments offer much-needed hope for patients suffering from chronic pain
A pain management specialist explains some of the new developments in pain treatment and why there’s…
ADHD brains present unique challenges, but the condition is highly treatable − a primary care nurse
Navigating life with ADHD or as a parent of a child with ADHD can be stressful. But as researchers learn…